Moored observations of turbulent mixing events in deep Lake Garda, Italy
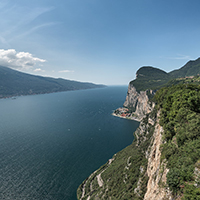
Submitted: 15 August 2020
Accepted: 26 October 2020
Published: 3 November 2020
Accepted: 26 October 2020
Abstract Views: 2767
PDF: 781
HTML: 13
HTML: 13
Publisher's note
All claims expressed in this article are solely those of the authors and do not necessarily represent those of their affiliated organizations, or those of the publisher, the editors and the reviewers. Any product that may be evaluated in this article or claim that may be made by its manufacturer is not guaranteed or endorsed by the publisher.
All claims expressed in this article are solely those of the authors and do not necessarily represent those of their affiliated organizations, or those of the publisher, the editors and the reviewers. Any product that may be evaluated in this article or claim that may be made by its manufacturer is not guaranteed or endorsed by the publisher.
Similar Articles
- Dimitar D. Doychev, Longitudinal recovery gradient of macroinvertebrates during different hydrological scenarios in a downstream river reach , Journal of Limnology: Vol. 82 (2023)
- Silvia Quadroni, Alex Laini, Francesca Salmaso, Livia Servanzi, Gaetano Gentili, Serena Zaccara, Paolo Espa, Giuseppe Crosa, Towards ecological flows: status of the benthic macroinvertebrate community during summer low-flow periods in a regulated lowland river , Journal of Limnology: Vol. 81 No. s2 (2022): Effects of water level management on lake littorals and downstream river areas
- Maciej Karpowicz, Biodiversity of microcrustaceans (Cladocera, Copepoda) in a lowland river ecosystem , Journal of Limnology: Vol. 76 No. 1 (2017)
- Rosario Mosello, An archive for the history of limnology in Verbania Pallanza (Northern Italy) , Journal of Limnology: Vol. 80 No. 3 (2021): Celebratory Issue - 80th Anniversary of the Journal of Limnology
- Johann P. Müller, David Laloi, Claude Yéprémian, Cécile Bernard, Florence D. Hulot, To flee or not to flee: detection, avoidance and attraction of profitable resources by Daphnia magna studied with olfactometer , Journal of Limnology: Vol. 72 No. 3 (2013)
- Maria Cristina Bruno, Mauro Carolli, Bruno Maiolini, Settling distances of benthic invertebrates in a sediment mobilization simulation in semi-natural flumes , Journal of Limnology: Vol. 75 No. 1 (2016)
- Emilia Rota, Marco Bartoli, Alex Laini, First time in Italy. Is the elusive aquatic megadrile Sparganophilus Benham, 1892 (Annelida, Clitellata) accelerating its dispersal in Europe? , Journal of Limnology: Vol. 73 No. 3 (2014)
- Nico SALMASO, Rosario MOSELLO, Letizia GARIBALDI, Fabio DECET, Maria Cristina BRIZZIO, Paolo CORDELLA, Vertical mixing as a determinant of trophic status in deep lakes: a case study from two lakes south of the Alps (Lake Garda and Lake Iseo) , Journal of Limnology: Vol. 62 No. s1 (2003): Residence Time in Lakes: Science, Management, Education
- Marina Vilenica, Mladen Kerovec, Ivana Pozojević, Zlatko Mihaljević, Odonata assemblages in anthropogenically impacted lotic habitats , Journal of Limnology: Vol. 80 No. 1 (2021)
- Sebastian Lenz, Uta Gruenert, Juergen Geist, Michael Stiefel, Maren Lentz, Uta Raeder, Calcite production by the calcifying green alga Phacotus lenticularis , Journal of Limnology: Vol. 77 No. 2 (2018)
<< < 33 34 35 36 37 38 39 40 41 42 > >>
You may also start an advanced similarity search for this article.