Modelling physical and ecological processes in medium-to-large deep European perialpine lakes: a review
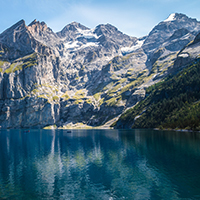
Accepted: 5 October 2021
HTML: 123
All claims expressed in this article are solely those of the authors and do not necessarily represent those of their affiliated organizations, or those of the publisher, the editors and the reviewers. Any product that may be evaluated in this article or claim that may be made by its manufacturer is not guaranteed or endorsed by the publisher.
Authors
In this paper we review a significant sample of the modelling studies carried out on medium-to-large deep European perialpine lakes (MLDEPLs). The reviewed bibliographic corpus was obtained querying Elsevier’s Scopus® database with a tailored search string on 8 January 2021. Results were filtered, accepting only journal papers written in English dealing with natural lakes having surface area > 10 km2. A list of 75 works was obtained, published between 1986 and 2021. Most studies have been carried out on Swiss lakes (44 out of 75 papers), Lake Geneva being the most investigated environment. A significant positive correlation was found between lake surface area and volume and the number of dedicated papers, suggesting that scientific attention is higher for environments characterised by large dimensions and relevant socio-economic interests. Both the number of papers and their citation count have experienced an exponential growth in time, pointing to a rising interest in quantitative modelling applications, but also to the increasing availability and ease of use of numerical modelling tools. Among the 75 selected papers, 55 employ a hydrodynamic driver, used alone or coupled with an ecological module, while the remnant 20 works adopt an ecological-only model. Among the papers employing hydrodynamic models, the use of three-dimensional (3D) drivers is surprisingly slightly more frequent (28 papers) than that of one-dimensional (1D) ones (26 papers), with most 3D applications having been published in the last 2011-2020 decade (24 papers). This reflects the interest on the hydrodynamic processes leading to the observed spatial heterogeneities in the biochemical properties of the MLDEPLs. However, coupling of ecological modules with 3D hydrodynamic drivers, to directly simulate these phenomena, is still restricted (2 papers) compared to that of 1D hydrodynamic drivers (8 papers), due to calibration and computational difficulties, which could be strongly reduced by future research achievements. Nevertheless, 1D models allow performing long-term prognoses considering multiple climate change and watershed management scenarios, due to their much smaller computational burden. The largest group of works dealing with ecological-only models (6 papers) is dedicated to applications of phosphorus budget models, which can above all be used to forecast variations in lake productivity in response to changes in the availability of the limiting nutrient.
Graphical abstractHow to Cite

This work is licensed under a Creative Commons Attribution-NonCommercial 4.0 International License.
Similar Articles
- Caterina Maria Antognazza, Silvia Quadroni, Isabella Vanetti, Vanessa De Santis, Giuseppe Crosa, Serena Zaccara, The increasing spread of the European barbel in the Italian large lowland rivers is threatening the native species , Journal of Limnology: Vol. 81 No. s2 (2022): Effects of water level management on lake littorals and downstream river areas
- Shengxing Long, Paul B. Hamilton, Yang Yang, Jianrong Ma, Ondhoro C. Chobet, Chuan Chen, Anzhi Dang, Zhiwei Liu, Xian Dong, Jiangan Chen, Multi-year succession of cyanobacteria blooms in a highland reservoir with changing nutrient status, Guizhou Province, China , Journal of Limnology: Vol. 77 No. 2 (2018)
- Feng Li, Gang Yang, Yonghong Xie, Xinsheng Chen, Zhengmiao Deng, Jiayu Hu, Competition between two wetland macrophytes under different levels of sediment saturation , Journal of Limnology: Vol. 74 No. 3 (2015)
- Fernando W. Bernal-Brooks, José J. Sánchez Chávez, Luis Bravo Inclán, Rubén Hernández Morales, Ana K. Martínez Cano, Owen T. Lind, Laura Dávalos-Lind, The algal growth-limiting nutrient of lakes located at Mexico’s Mesa Central , Journal of Limnology: Vol. 75 No. s1 (2016): Proceedings of the 6th National Congress of Limnology
- Laura Sartori, Sergio Canobbio, Riccardo Cabrini, Riccardo Fornaroli, Valeria Mezzanotte, Macroinvertebrate assemblages and biodiversity levels: ecological role of constructed wetlands and artificial ponds in a natural park , Journal of Limnology: Vol. 74 No. 2 (2015)
- Amanda Valois, Keith Somers, Chantal Sarrazin-Delay, Wendel (Bill) Keller, Are bioassessments based on the reference condition approach affected by rapid approaches to sample collection and processing? , Journal of Limnology: Vol. 75 No. 2 (2016)
- María del Carmen Hernández, Javier Alcocer, Luis A. Oseguera, Elva Escobar, Profundal benthic invertebrates in an oligotrophic tropical lake: different strategies for coping with anoxia , Journal of Limnology: Vol. 73 No. 2 (2014)
- Elzbieta Dumnicka, Kamil Najberek, Valeria Lencioni, Oligochaete distribution in alpine freshwaters: not a mere question of altitude , Journal of Limnology: Vol. 82 (2023)
- Carlos Martinez-Sanz, Camino Fernandez-Alaez, Francisco Garcia-Criado, Richness of littoral macroinvertebrate communities in mountain ponds from NW Spain: what factors does it depend on? , Journal of Limnology: Vol. 71 No. 1 (2012)
- Marco Pilotti, Stefano Simoncelli, Giulia Valerio, Computing the transport time scales of a stratified lake on the basis of Tonolli’s model , Journal of Limnology: Vol. 73 No. 3 (2014)
<< < 13 14 15 16 17 18 19 20 21 22 > >>
You may also start an advanced similarity search for this article.
-
Laura M. V. Soares, Olivia Desgué‐Itier, Cécilia Barouillet, Céline Casenave, Isabelle Domaizon, Victor Frossard, Nelson G. Hairston, Andrea Lami, Bruno J. Lemaire, Georges‐Marie Saulnier, Frédéric Soulignac, Brigitte Vinçon‐Leite, Jean‐Philippe JennyLimnology and Oceanography Letters : 2024
-
Diego CopettiResources : 2023
-
Andrea Fenocchi, Fabio Buzzi, Claudia Dresti, Diego CopettiEcological Indicators : 2023